Research
We are an interdisciplinary lab, working at the interface of Biochemistry, Cell Biology, and Physics. Our work is driven by a sense of curiosity, fascination, and discovery. We study how biological systems—from molecules to organisms—achieve remarkable complexity through self-assembly, where local interactions give rise to emergent order. By combining quantitative experiments, theory, and computational modeling, we aim to uncover universal principles governing self-organization across scales.
Our lab's research is broadly organized across the following two themes:

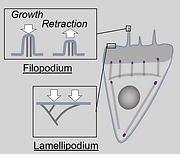
Theme A: Molecular Self-assembly
Multicomponent mechanochemical regulation of actin dynamics
Cellular actin dynamics are essential in key processes such as cell migration, wound healing, division, and endocytosis. Living cells rapidly assemble and remodel their actin cytoskeleton in response to external signals to move, change shape, and reorganize their internal architecture. Cells can locally tune actin dynamics to meet the demands of specific biological processes, but how is this achieved in the crowded, heterogeneous cytoplasm? The central hypothesis driving our research is that intracellular actin dynamics emerges from an interplay between multicomponent biochemical factors (e.g., proteins) and mechanical forces. By reconstituting minimal actin networks and probing their dynamics in vitro, we seek to decode the rules of self-organization that enable actin dynamics in vivo
Theme B: Organismal Self-Assembly
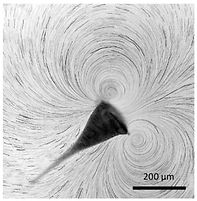
How did the first unicellular organisms transition to cooperative multicellular-like states? Using the ciliate Stentor as a model, we are investigating the role of physical forces — particularly hydrodynamics — in driving the emergence of collective behavior. Our recent work (Nature Physics, 2025) revealed that Stentor colonies self-organize dynamically, with hydrodynamic coupling between neighboring individuals enhancing feeding currents asymmetrically. This suggests that fluid mechanics could have been an early selective pressure favoring multicellularity, even before the evolution of permanent cell-cell adhesions. By integrating high-speed live imaging, mathematical modeling, and evolutionary biophysics, we are exploring how transient physical interactions enable functional cohesion in colonies, bridging the gap between simple unicellular and complex multicellular systems.
Our biophysical toolbox


Total internal reflection microscopy (2 Nikon E-TIRFs + 1 iLas ring TIRF scopes)
Microfluidics (Automated flow control systems from Fluigent)
Optical tweezers (Lumicks C-Trap confocal setup)
Magnetic Tweezers
Multispectral single-molecule imaging
High-speed imaging